In a world striving to reduce the environmental impact of energy production, intelligent Energy Management Systems (EMS) can play a key role in increasing energy production and consumption efficiency, and facilitate the integration of renewable energy sources within conventional generation systems. EMS can locally handle the supply of different energy services, controlling renewable and conventional distributed energy resources, storage systems, and dispatchable loads to exploit internal synergies and minimise operation cost and / or environmental impact. Our objective is to devise the architecture of advanced multi-energy microgrids, and develop dispatch optimisation algorithms that can ensure optimal and reliable performances.
The GECOS group has developed an inter-departmental laboratory to test and analyse microgrids performance. Check out our Multi-Good Micro-Grid Laboratory (MG2Lab)
Cogeneration, trigeneration and multigeneration
GECOS group has a long history in designing distributed generation systems for the combined production of electricity, heat and cooling.
Development and testing of innovative distributed energy devices
Techno-economic assessment of complex systems and development of user-friendly tools for energy assessment (CHP design web app)
Advanced optimisation algorithms and control logic to identify the best operational strategy in complex systems
Collaboration with energy services providers to deploy and field test our solutions
Recently, our know-how has extended to multi-energy systems, where generic goods that can represent services, energy fluxes or actual products are exchanged and converted into one another within a local microgrid.
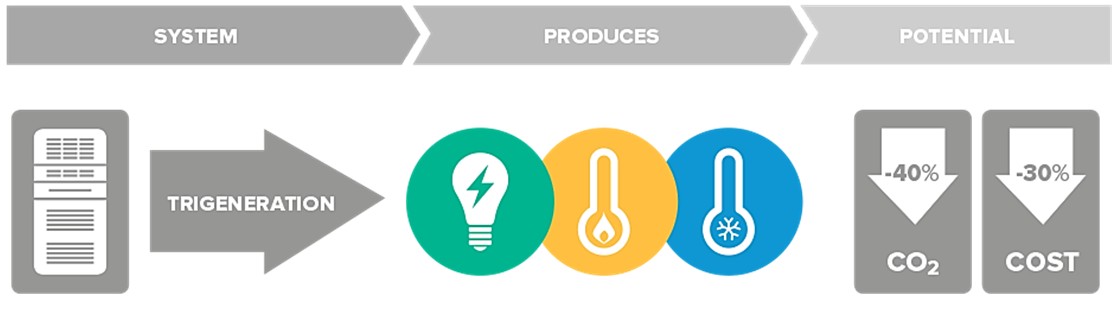
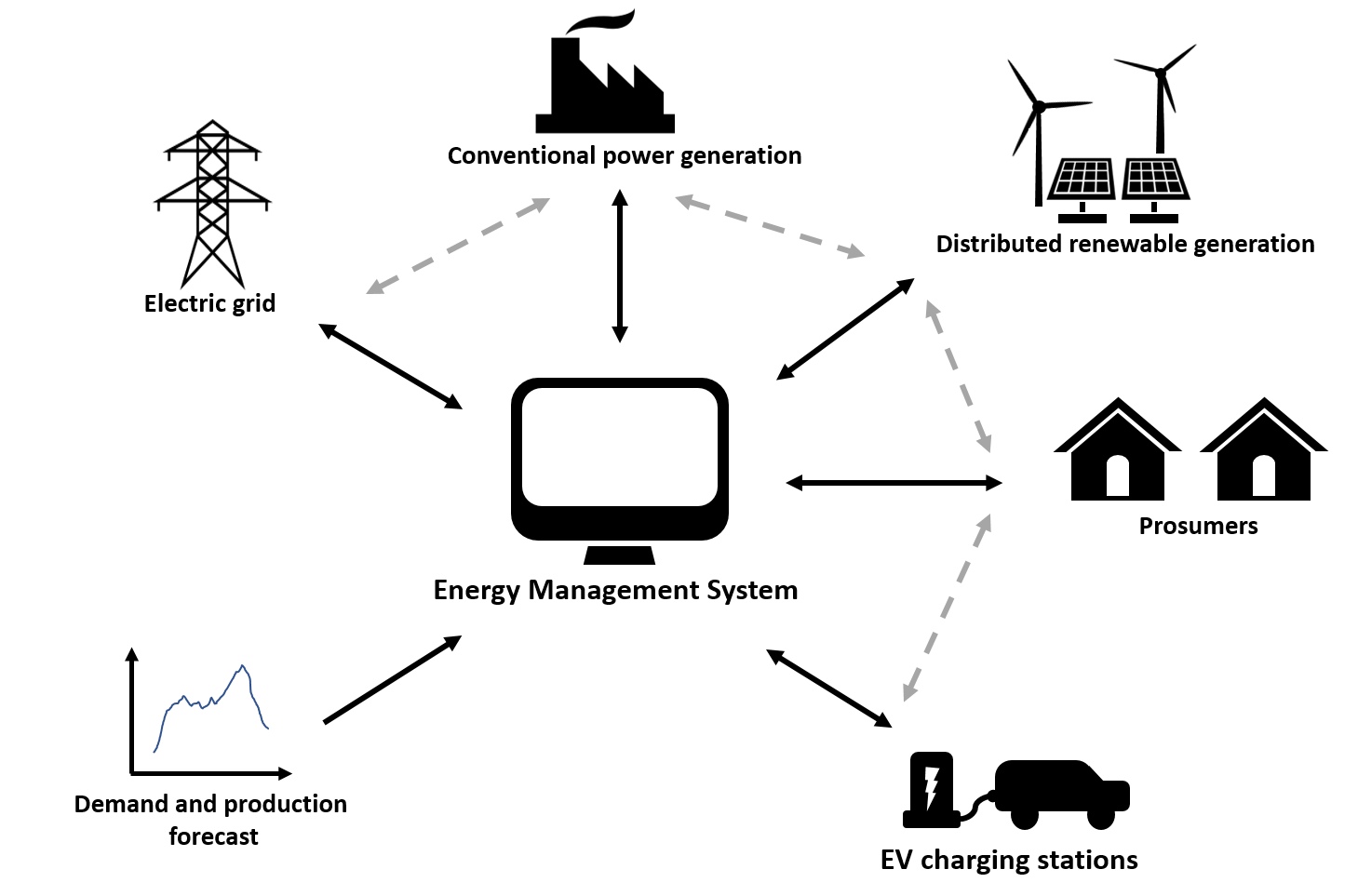
The future of electricity is digital!
The increasing electrification of our society (involving transports, HVAC systems, and most of domestic and non-domestic appliances), and the diffuse digitalisation of control systems, offers great opportunities for integrated energy production and consumption management. Our research focuses on:
Integration of Electric Vehicles (EV) recharge stations in multi-energy microgrids
Collective management of distributed demand (aggregators) and generation (virtual power plants)
Home automation and high-penetration renewable energy domestic systems
Microgrids for rural development
GECOS group has been active for many years in the field of rural electrification and development focusing on:
Design and operation optimisation of islanded microgrids for the electrification of remote locations
Exploitation of local resources (biomass, solar, wind) to provide electricity and services, like water potabilization
Strategic evaluation of investments in microgrids considering competition with grid-extension planning and electric demand evolution
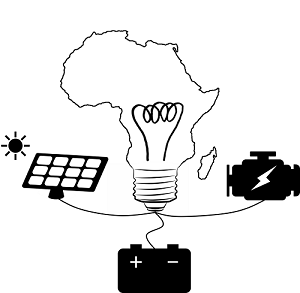
For further information, please contact Prof. Giampaolo Manzolini (giampaolo.manzolini@polimi.it).
Want to know more about the high efficiency cogeneration regulatory framework in Italy?
Have a look at this incentives guideline summary proposed by GECOS.
For additional information, please contact Ing. Nicola Fergnani (nicola.fergnani@polimi.it).
Related Projects
Recent publications
Sorry, no publications matched your criteria.